Biologists build proteins that avoid crosstalk with existing molecules
Engineered signaling pathways could offer a new way to build synthetic biology circuits
23 Oct 2019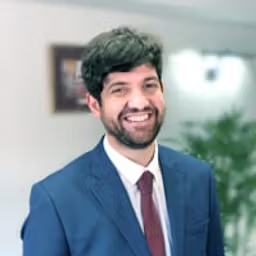
Inside a living cell, many important messages are communicated via interactions between proteins. For these signals to be accurately relayed, each protein must interact only with its specific partner, avoiding unwanted crosstalk with any similar proteins.
A new MIT study sheds light on how cells are able to prevent crosstalk between these proteins, and also shows that there remains a huge number of possible protein interactions that cells have not used for signaling. This means that synthetic biologists could generate new pairs of proteins that can act as artificial circuits for applications such as diagnosing disease, without interfering with cells’ existing signaling pathways. “Using our high-throughput approach, you can generate many orthogonal versions of a particular interaction, allowing you to see how many different insulated versions of that protein complex can be built,” says Conor McClune, an MIT graduate student and the lead author of the study. In the new paper, which appears today in Nature, the researchers produced novel pairs of signaling proteins and demonstrated how they can be used to link new signals to new outputs by engineering E. coli cells that produce yellow fluorescence after encountering a specific plant hormone. Michael Laub, an MIT professor of biology, is the senior author of the study. Other authors are recent MIT graduate Aurora Alvarez-Buylla and Christopher Voigt, the Daniel I.C. Wang Professor of Advanced Biotechnology.
In this study, the researchers focused on a type of signaling pathway called two-component signaling, which is found in bacteria and some other organisms. A wide variety of two-component pathways has evolved through a process in which cells duplicate genes for signaling proteins they already have, and then mutate them, creating families of similar proteins. “It’s intrinsically advantageous for organisms to be able to expand this small number of signaling families quite dramatically, but it runs the risk that you’re going to have crosstalk between these systems that are all very similar,” Laub says. “It then becomes an interesting challenge for cells: How do you maintain the fidelity of information flow, and how do you couple specific inputs to specific outputs?” Most of these signaling pairs consist of an enzyme called a kinase and its substrate, which is activated by the kinase. Bacteria can have dozens or even hundreds of these protein pairs relaying different signals.
About 10 years ago, Laub showed that the specificity between bacterial kinases and their substrates is determined by only five amino acids in each of the partner proteins. This raised the question of whether cells have already used up, or are coming close to using up, all of the possible unique combinations that won’t interfere with existing pathways. Some previous studies from other labs had suggested that the possible number of interactions that would not interfere with each other might be running out, but the evidence was not definitive. The MIT researchers decided to take a systematic approach in which they began with one pair of existing E. coli signaling proteins, known as PhoQ and PhoP, and then introduced mutations in the regions that determine their specificity.
This yielded more than 10,000 pairs of proteins. The researchers tested each kinase to see if they would activate any of the substrates and identified about 200 pairs that interact with each other but not the parent proteins, the other novel pairs, or any other type of kinase-substrate family found in E. coli. “What we found is that it’s pretty easy to find combinations that will work, where two proteins interact to transduce a signal and they don’t talk to anything else inside the cell,” Laub says. He now plans to try to reconstruct the evolutionary history that has led to certain protein pairs being used by cells while many other possible combinations have not naturally evolved.
This study also offers a new strategy for creating new synthetic biology circuits based on protein pairs that don’t crosstalk with other cellular proteins, the researchers say. To demonstrate that possibility, they took one of their new protein pairs and modified the kinase so that it would be activated by a plant hormone called trans-zeatin and engineered the substrate so that it would glow yellow when the kinase activated it. “This shows that we can overcome one of the challenges of putting a synthetic circuit in a cell, which is that the cell is already filled with signaling proteins,” Voigt says. “When we try to move a sensor or circuit between species, one of the biggest problems is that it interferes with the pathways already there.” One possible application for this new approach is designing circuits that detect the presence of other microbes. Such circuits could be useful for creating probiotic bacteria that could help diagnose infectious diseases. If adapted for use in human cells, this approach could also help researchers design new ways to program human T cells to destroy cancer cells. This type of therapy, known as CAR-T cell therapy, has been approved to treat some blood cancers and is being developed for other cancers as well. Although the signaling proteins involved would be different from those in this study, “the same principle applies in that the therapeutic relies on our ability to take sets of engineered proteins and put them into a novel genomic context, and hope that they don’t interfere with pathways already in the cells,” McClune says.
Register for your free SelectScience membership today to receive the latest editorial articles and technology news direct to your inbox>>