Could ‘Onion’ Vesicles Revolutionize Drug Delivery?
19 Jun 2014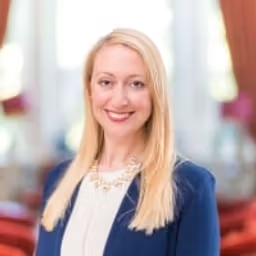
A research group led by Professor Virgil Percec, of the Department of Chemistry in Penn’s School of Arts & Sciences, has successfully replicated the vesicle-like properties of a cell membrane. Such a structure opens up possibilities of releasing drugs over longer periods of time, with a new dose in each layer, or even putting a cocktail of drugs in different layers so each is released in sequence.
A research group led by Professor Virgil Percec, of the Department of Chemistry in Penn’s School of Arts & Sciences, has successfully replicated the vesicle-like properties of a cell membrane. Such properties keep a cell’s repository of DNA and protein-making machinery stable and secure from invaders and toxins. Scientists have previously attempted to replicate these properties in a basic vesicle, but they have lacked consistency in size and stability.
The University of Pennsylvania researchers have shown in their study, published in Proceedings of the National Academy of Sciences, that a certain kind of dendrimer (called an amphiphilic Janus dendrimer), a molecule that features tree-like branches, offers a simple way of creating vesicles and tailoring their diameter and thickness. Moreover, these dendrimer-based vesicles self-assemble with concentric layers of membranes, much like an onion.
The researchers found that after dissolving those molecules in an alcohol solution and injecting them into water they formed stable, evenly sized vesicles.
By altering the concentration of the dendrimers suspended within, the researchers have shown that they can control the number of layers, and thus the diameter of the vesicle, when the solution is injected in water. Such a structure opens up possibilities of releasing drugs over longer periods of time, with a new dose in each layer, or even putting a cocktail of drugs in different layers so each is released in sequence.
Membrane versatility
Cell membranes are made of two layers of molecules, each of which has a head that is attracted to water and a tail that is repelled by it. These bilayer membranes self-assemble so that the hydrophilic heads of the molecules of both layers are on the exterior, facing the water that is in the cell’s environment as well as the water encapsulated inside.
Stripping out the additional proteins and sugars that cells naturally have in their membranes leaves just a double-walled bubble that can be stuffed with drugs or other useful content.
“The problem,” Percec said, “is that once you remove the proteins and the other elements of a real biological membrane, they are unstable and don't last for a long time. It's also hard to control their permeability and their polydispersity, which is how close together in size they are. The methodologies for producing them are also complicated and expensive.”
Research in this field has thus been focused on finding new chemistries to replace the fatty molecules that normally make up a vesicle’s bilayer.
Structural biology technology
Not all cells are content with just a single bilayer, however. Some biological systems, such as gram-negative bacteria and the myelin sheaths that cover nerves, have multiple concentric bilayers. Having a model system with that arrangement could provide some fundamental insights to these real-world systems, and the added stability of extra layers of padding would be a useful trait in clinical applications. However, methods for producing vesicles with multiple bilayers remained elusive.
“The only way it has been achieved in the past was through a complicated mechanical process, which was a dead end,” Percec said. “This was not a viable option for mass-producing multilayered vesicles, but with our library of amphiphilic Janus dendrimers, we were lucky to find some molecules that have, in their chemicals, instructions needed to self-assemble into these very beautiful structures.”
Using various techniques, including high performance liquid chromatography (HPLC), Nuclear Magnetic Resonance (NMR) and Maldi-TOF Mass Spectrometry, the team tested different dendrimers with different organic solvents, and found they could produce these onion-like vesicles and control the number of layers they contained. By changing the concentration of the dendrimers in the solvent, they could produce vesicles with as many as 20 layers when that solution was introduced to water. And because the layers are consistently spaced, the team could control the overall size of the vesicles by predicting the number of layers they could contain.
Drug encapsulation
To actually see the multilayered structure of their vesicles, the researchers used a technique known as cryogenic transmission electron microscopy, or CryoTEM using a Joel 2100 TEM equipped with a cryogenic holder. This technique can take pictures of objects floating in aqueous solution at the nanoscale. To keep the fluid-floating vesicles in frame, the team flash-froze the samples, locking them in amorphous ice that was free of damaging ice crystals.
Professor Percec told SelectScience, “It's too early to predict the capabilities of the vesicles with regards cancer therapeutics, but with the vesicles characterized, a host of clinical applications are possible, including hydrophilic and hydrophobic drugs, nucleic acids and proteins.”
One of the more enticing applications is encapsulating drugs in these vesicles. Many drugs are not water-soluble, so they need to be packaged with some other chemistry to allow them to flow through the bloodstream. The additional stability of multiple bilayers makes these onion vesicles an attractive option, and their unique structure opens the door to next generation nanomedicine.
“If you want to deliver a single drug over the course of 20 days,” Percec said, “you could think about putting one dose of the drug in each layer and have it released over time. Or you might put one drug in the first layer, another drug in the second and so on. Being able to control the diameter of the vesicles may also have clinical uses; target cells might only accept vesicles of a certain size.”
Based on an article from Penn University and interview with Professor Percec
Image credit: Penn University