Improving hydrogen storage in the quest to reach ‘net zero’
Award-winning UK scientist Dr. Lui Terry discusses how his pioneering work on nanoconfinement could help advance the global transition to cleaner energy
17 Aug 2020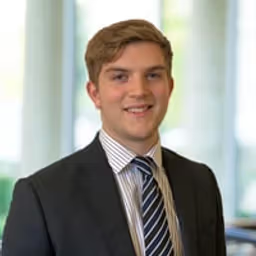
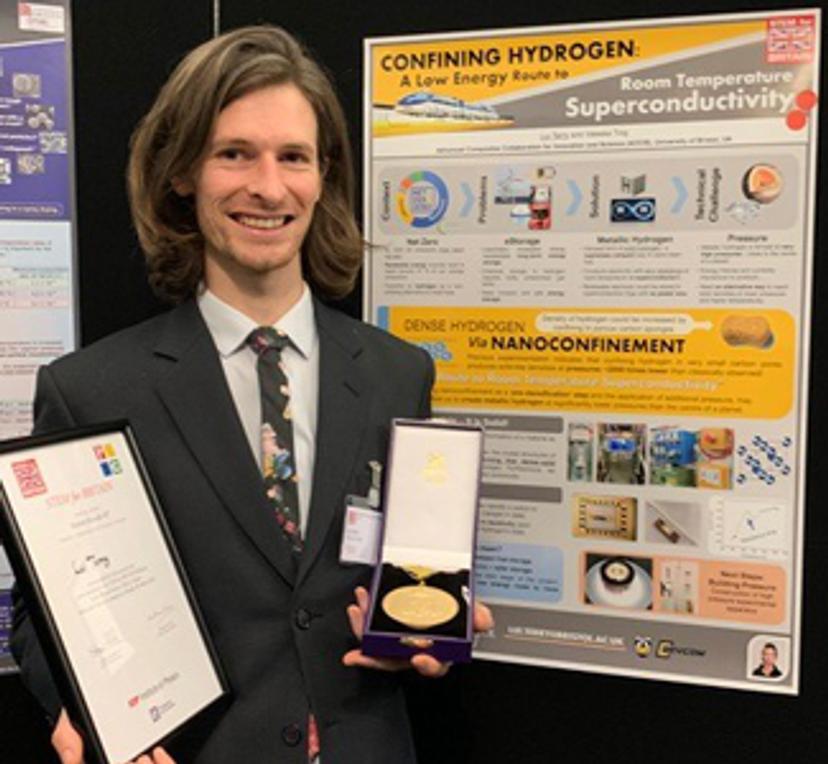
In this article, we hear from the winner of the UK Parliament's Cavendish Gold Medal for Physics and postdoctoral fellow at the University of Bristol, Dr. Lui Terry, about his research on improving hydrogen storage by exploiting nanoconfined environments. Here, our former SelectScience colleague discusses how net-zero greenhouse gas (GHG) emissions can be achieved, the current challenges scientists face when storing hydrogen and his hopes for the future.
What are the aims of your research?
My research focuses on how hydrogen behaves in nanoconfined environments such as porous materials and how we can exploit nanoconfined behavior to improve hydrogen storage and attempt to reach the holy grail of physics – room temperature superconductivity.
To put this into context, the UK has legislated that by 2050 its emissions must reach net zero. To do this, the Committee on Climate Change has outlined renewable energy sources to produce around 57% of our energy production including a transition to hydrogen as a non-polluting alternative to fossil fuels.
What are the current challenges of reaching net-zero?
The main issue is that the intermittent use of renewable energy necessitates long-term energy storage, such as chemical storage in hydrogen. However, hydrogen is currently stored as a gas, which requires bulky pressurized tanks – bringing in questions of efficient storage and safety for transport.
How can we improve energy storage?
One solution is to produce metallic hydrogen, the densest form of solid hydrogen — a supremely compact way to store clean fuel. Even more impressively, it conducts electricity with zero resistance at room temperature, a superconductor. In this way, renewable electricity could be stored in superconductive rings with no power loss and supply instantaneous high-power output required for industry.
Metallic hydrogen is the densest form of solid hydrogen - a supremely compact way to store clean fuel
Dr. Lui Terry University of Bristol
However, metallic hydrogen is classically formed at very high pressures – close to the center of a planet. Thus, it is energy intense and currently impractical to produce, but it is predicted to be metastable like diamonds. Therefore, on removal of the pressure, they remain solid.
How can nanoconfinement help?
Here in Bristol, we are developing an alternative way to increase density at much lower pressures and higher temperatures — we work on the effects of nanoconfinement. Recent work has indicated that nanoconfined hydrogen reaches solid-like densities at pressures ~ 2000 times lower than would be expected outside the pores. Thus, nanoconfinement can cause a spontaneous densification effect allowing us to manipulate the phase diagram to produce dense matter at temperatures and pressures not classically possible.
Metallic hydrogen could revolutionize energy storage’
Dr. Lui Terry University of Bristol
What do you see for your research in the future?
My research objective is to understand the mechanisms of nanoscale confinement effects on hydrogen as well as other gas mixtures, and exploit them to produce extremely dense phases matter. We hope to use nanoconfinement as ‘a pre-densification’ step to significantly lower the pressures to reach room temperature superconductivity which could revolutionize energy storage.
By integration of superconductive storage rings in the national grid, excess intermittent renewable energy could be stored without loss of power. Furthermore, identification of further solid phases at higher temperatures and lower pressures would lead to more efficient, compact, and safer hydrogen storage for transport. I believe over the next decade we will see some very big changes in the energy industry, and I hope that my research will play its part in this.
Want the latest science news straight to your inbox? Become a SelectScience member for free today>>