Monitoring electronic excitations in organic semiconductors with ultrafast optical pump-probe spectroscopy
Watch this on-demand webinar to learn more about fs-pump white-light probe spectroscopy, from experimental challenges to how to ensure reliable results
5 Nov 2021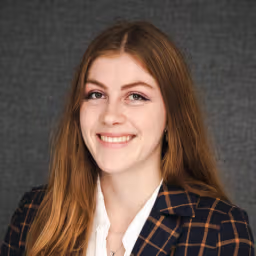
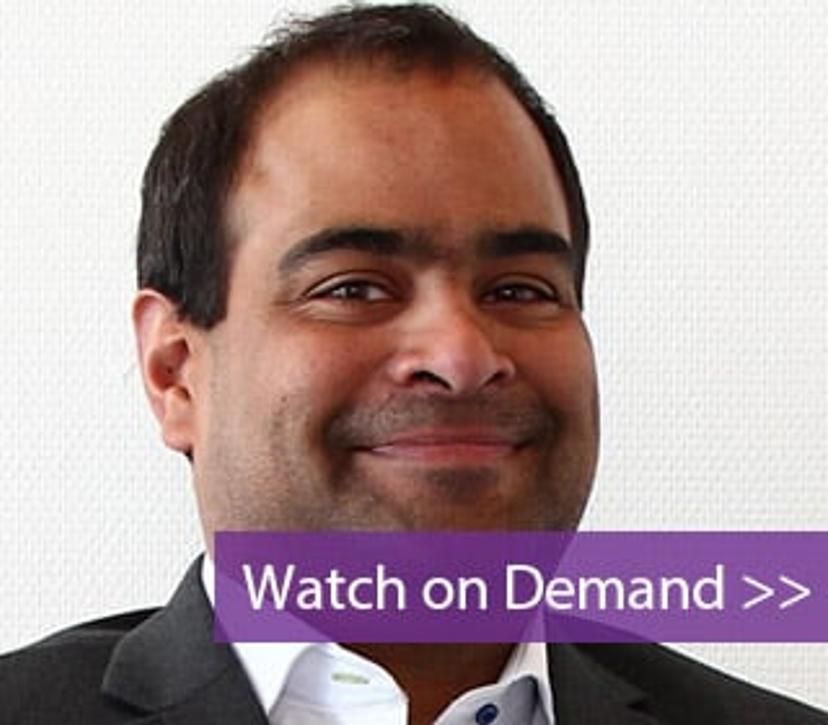
Polarization, time, and energy-resolved optical pump-probe spectroscopy is a well-established tool to monitor charge-carrier dynamics on ultrafast timescales. The general idea is that an optical excitation changes the linear response (“absorption”) properties of a sample which can be monitored optically, in a contactless, non-invasive fashion.
In this free SelectScience® webinar, now available on demand, Dr. Sangam Chatterjee, Professor at the Institute of Experimental Physics I at Justus-Liebig-University (JLU) Gießen, provides a comprehensive overview of ultrafast optical pump-probe spectroscopy. Chatterjee discusses strengths and limitations compared to alternative methods, the technical requirements for a successful experiment, how it may be implemented with spatial, temporal, energy, and polarization resolution, and more.
Watch on demandRegister now to watch the webinar on demand, and read on for highlights from the Q&A discussion:
How do you correct for photoluminescence in pump-probe data?
SC: There's always photoluminescence when you excite a sample with a pump. It can be intrinsic or extrinsic. It can be scattered light which, strictly speaking, isn’t photoluminescence. But when the pump is on, there's some light in connection with the pump going into the sample that's not part of your pump and probe data. Let's call this photoluminescence for simplicity.
We have to correct for this. If you work with a CCD camera, the background is typically something like readout noise or dark current from the detectors which gives you a signal even if you block the light. This is what you subtract from the transmitted light.
Now, you have the light with pump on and probe on, and you actually subtract the photoluminescent spectrum which contains all the scattered light, all the resonant Raman scattering, the Rayleigh scattering and the photoluminescence that you use instead of your dark electronic background or dark picture, which you would take for the probe only.
You have to do that before you divide the respective spectra and take the logarithm, or just don't take the logarithm if you want to look at true transmissions. This way, you can account for the photoluminescence. But how constant is this? Do you have to take the spectrum repeatedly, or can you just take this once? This depends on the system stability. You need to make sure your system is very stable, and that nobody moves around, and you don't have any air conditioning issues, etc. A lot of the time these things are very important.
Every time you have a different pump-probe delay, you take a new photoluminescence reference spectrum. If you're confident that your system is very stable or your run is very short, you can just take this once. Everything is going to be corrected for.
How efficient is the time resolve in photoluminescence spectroscopy?
SC: The efficiency can be looked at in two ways. The first is time-resolved total luminescence. The way we do that is using a street camera. Street cameras are basically the fastest detectors you can buy with good imaging resolution. The fastest time resolution we have at hand is something like 600 femtoseconds. This resolution is awkward to work with because if you wave your hand in front of the beam, you will see the airflow in the position and time when the photoluminescence hits the photocathode.
If efficiency is related to time resolution, the fastest street cameras I'm aware of currently on the market are something like 200 femtoseconds in a single shot. We have one which is running synchronously to the laser, which gives us something like 600, 700 femtoseconds in the visible.
We have a near-infrared street camera, which was discontinued very recently, that has about 20 picoseconds time resolution. That's also a single-shot camera. But it goes to 1.5 microns, and the faster ones are typically only in the visible range, something like 190 nanometers to 870 nanometers or so.
If efficiency is related to the number of photons that you have, these, in principle, have single-photon sensitivity because in the end, it's a photomultiplier. This means you can go to single photons and you can do single-photon counting. Very low light levels are required here because you have to ensure that you only have one photon per pixel, per pulse at most.
As you work with small spots, do you have polarization issues and light level issues?
SC: Yes, we do have light level issues, and one has to be careful that the probe is actually linear on the sample. We cannot do single-shot measurements, because if we do single shots, meaning only detecting one laser shot per readout of the camera, we don't have high enough light levels across this broad spectrum, so we average typically a few hundreds of shots or more.
You have to be careful, if you don't time the camera on your laser, that you have enough photons in there and you don't cause any non-linearities.
We do this by averaging, so if you only average nine pulses, then you really have to be careful about your timing, because the difference between eight, nine, and ten pulses is something like 10% or 12%, depending on how you calculate it, in the number of photons you have available. That works well if you self-reference because then your spectra will just jump up and down in amplitude. But that is not too bad if you make sure it's always the same pulse you look at.
If you don't do that, then you better take more pulses because if you have 1,000 pulses in there, 1,000 or 1,001, that is typically only very little change. You should also be careful in these experiments to fill the wells of your cameras, because you don't want to be limited by the readout noise, or too low light levels, or crosstalk in cameras, or smear if you don't shutter – and shuttering can be tedious because it shakes the experiment if you use a mechanical shutter.
Light level is always an issue. Small spots and polarization do work if you have correct optics. You can buy maintaining microscope objectives if you want to use microscope objective polarization. We very often use mirrors. That's a little bit trickier. You must make sure that your angles of incidence are either 45 degrees because then you don't do too many bad things, because you just could flip in the phase, or you'd use close-to-normal incidents.
What you have to make sure is that, at the point of the sample, the polarization is what you want it to be, so you can check it there using a polarimeter, or a polarizer if you do the control test.
Then you can use things like Babinet compensators, or Soleil-Babinets, which don't really distort the beam path, to make sure that you have the polarization that you desire.
To learn more about ultrafast optical pump-probe spectroscopy, watch the webinar on demand >>
SelectScience runs 10+ webinars a month across various scientific topics, discover more of our upcoming webinars>>