Progressing precision medicine through enhanced proteomics analyses
Leveraging the molecular insights derived from blood-based biomarkers creates a unique understanding of an individual's molecular health-state
22 Apr 2024
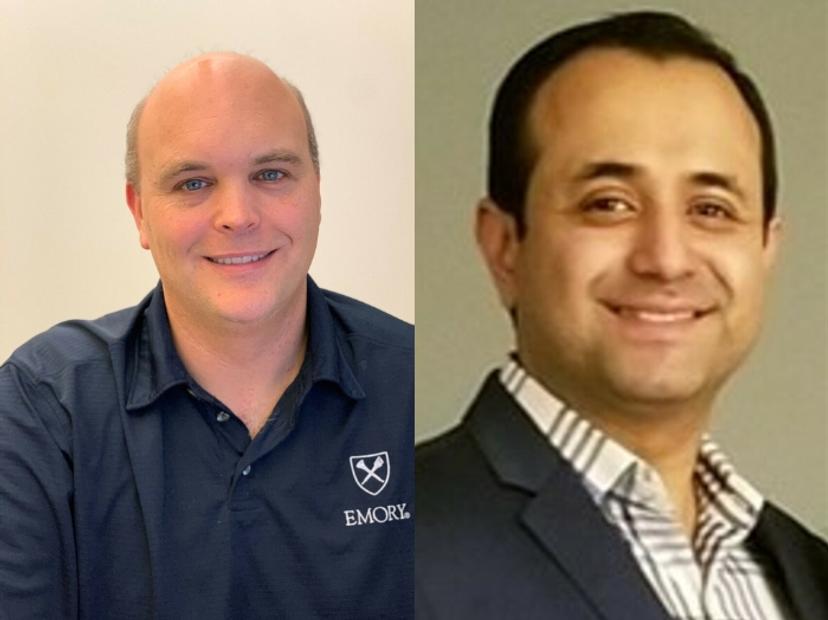
Blood plasma is a readily available, easily obtainable and rich source for biomedical research and biomarker discovery as it connects to almost all tissues in the body, thereby reflecting an individual’s health and disease state.
Advancements in robust and scalable proteomics analysis of plasma provide new clinically relevant avenues for biomedical research, ushering in a new era of precision medicine, where diagnosis and treatments are personalized according to the patient’s molecular profile. Leveraging the molecular insights derived from blood-based biomarkers creates a unique understanding of an individual's molecular health-state with potential to predict a patient's response to certain therapies.
Why plasma proteomics?
The exploration of blood proteins dates back to the mid-20th century, with early studies focused on understanding the basic composition of plasma. The true revolution began in the late 20th and early 21st centuries with the emergence of high-throughput technologies. These innovations enabled researchers to delve deeper into the complex and dynamic nature of plasma proteins. Interest grew in establishing the significance of proteomics as a tool for developing biomarkers and informing drug discovery.
The Human Proteome Organization (HUPO) played a key role by initiating large-scale international projects such as the Human Plasma Proteome Project (HPPP), to systematically map the entire plasma proteome. As mass spectrometry (MS) techniques continued to evolve, the field of plasma proteomics witnessed significant strides in robustness, sensitivity, speed, and accuracy. This progress paved the way for the identification of novel biomarkers, contributing to advancements in diagnostics and our overall understanding of human health.
The human plasma proteome is considered to be one of the most challenging proteomes to decipher as it covers a dynamic range of 1012 between the highest and lowest abundant proteins and contains proteins decorated with various post-translational modifications such as glycosylations.
The new gold standard for MS
The challenge in diagnosing and treating neurodegenerative diseases lies in their complex and multifaceted nature. Traditional diagnostic methods often rely on clinical symptoms and, in some cases, neuroimaging techniques like magnetic resonance imaging (MRI) or positron emission tomography (PET), or are determined by post-mortem tissue imaging. However, these approaches often have insufficient sensitivity or are incapable of detecting early-stage disease or providing prognostic or mechanistic insight.
While MS is an excellent tool for probing protein-ligand interaction and protein binding, MS alone lacks the ability to give definite measurements of the molecular interactions and downstream effects of those complexes. Trapped ion mobility spectrometry (TIMS) has emerged as a novel technique for the separation of peptides and proteins, especially those low abundant, difficult-to-isolate species involved in many diseases. TIMS involves an electrical gradient which prevents each ion from moving beyond a certain position in the TIMS tunnel, and the passage of the ions is defined by the ion’s shape in gas phase. This allows the selective release, fragmentation, identification, and quantification of ions from the TIMS tunnel in a process called parallel accumulation-serial fragmentation (PASEF). With TIMS and PASEF, the ion mobility measurements can be used to determine ion specific collisional cross section (CCS) values. The incorporation of CCS provides an additional fourth dimension to the analyses, which allows researchers to transition from 3D-proteomics (retention time, mass to charge (m/z) and tandem MS (MS/MS) fragment ion spectra) to 4D-proteomics. The addition of CCS values further increases the system’s selectivity, resulting in more reliable quantitation in complex samples.
The impact of CCS at The Roberts Lab
The Roberts Lab is a cutting-edge research facility based at the Emory University School of Medicine, in Atlanta, Georgia, using novel proteomics techniques and advanced technologies to better understand neurodegenerative disease. The lab has a comprehensive suite of analytical technologies and chromatography systems, plus a range of spectrometers, coupled to MS and MS/MS detectors for proteomics research. Rapid high-resolution matrix-assisted-laser-desorption/ionization (MALDI) imaging is used in conjunction with TIMS to give researchers the ability to accurately correlate measured results with morphological context, resolving the spatial distribution of proteins down to 5 µm lateral resolution. The result is a true spatial omics platform with widespread application.
An example of this in practice is in the team’s investigation of the role of amyloid beta in Alzheimer’s disease. Since MS alone can’t differentiate isomers with the same m/z, The Roberts Lab relies on MALDI Imaging combined with TIMS to separate isomeric molecules to understand the true spatial localization of the analyte. Combining TIMS and MALDI capabilities is currently the only method to differentiate isomers and resolve their spatial distributions. Using CCS values, the identity of an analyte can be resolved and validated with this additional quality criterion, because CCS-enabled software intelligently matches spatial MALDI-TIMS imaging data with omics results to add biomedically important morphological context to quantitative, isoform resolved proteomics data.
The next step for The Roberts Lab is to image amyloid beta in tissue to understand whether the isomers are localized exclusively around plaques, or uniformly distributed in the grey matter of the brain.
The next steps in early disease detection
The exceptional sensitivity and selectivity of 4D plasma proteomics enable the identification of more precise biomarkers, improvement of patient stratification, and development of methods to predict treatment response. Together, TIMS and PASEF hold the potential to transform clinical practice by providing more accurate and comprehensive data that can give improved insights into disease mechanisms and potential therapeutic targets, which opens new possibilities for improvements in early disease detection and the move towards personalized treatments.
This guest editorial article was written by Dr. Blaine Roberts, Associate Professor at Emory University and Dr. Shourjo Ghose, Application Scientist at Bruker Daltonics.
About the authors
Dr. Blaine Roberts is an Associate Professor in the Department of Biochemistry and Department of Neurology at Emory University. He obtained his Bachelor of Science in Chemistry at Montana State University and his PhD in Biochemistry and Biophysics from Oregon State University.
Dr. Shourjo Ghose received his PhD in Biochemistry from Montana State University and went on to do postdoctoral work at the Scripps Research Institute and gained a business certification from Harvard Business School. He joined Bruker as an application scientist in 2017 working on proteomics workflows on the timsTOF line of systems. He is presently responsible for managing the Bruker proteomics business in North America.