Scripps expert answers your questions on using NMR to study intrinsically disordered proteins
Prof. Jane Dyson discusses how IDPs are crucial for protein function and emphasizes the benefits of NMR as a technique for investigating these protein mechanisms
23 Mar 2020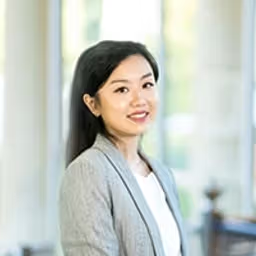
A large fraction of the cell's most vital functions comprises proteins that are disordered in regions crucial for the biological functions. Detailed studies of these proteins lead to a better understanding of protein folding and its functioning. Thanks to advances in nuclear magnetic resonance (NMR) instrumentation and methodology, an array of NMR tools is available for atomic resolution characterization of intrinsically disordered proteins (IDPs).
In this on-demand webinar, Prof Jane Dyson, from the Department of Integrative Structural and Computational Biology at the Scripps Research Institute, discusses the importance of intrinsic disorder in proteins for cell function and dysfunction. Professor Dyson explains how to apply high-resolution NMR spectroscopy to provide information at an atomic level, obtained in proteins' native state.
Read on for highlights from the live webinar Q&A session or register to watch the webinar at any time that suits you.
Watch Webinar Now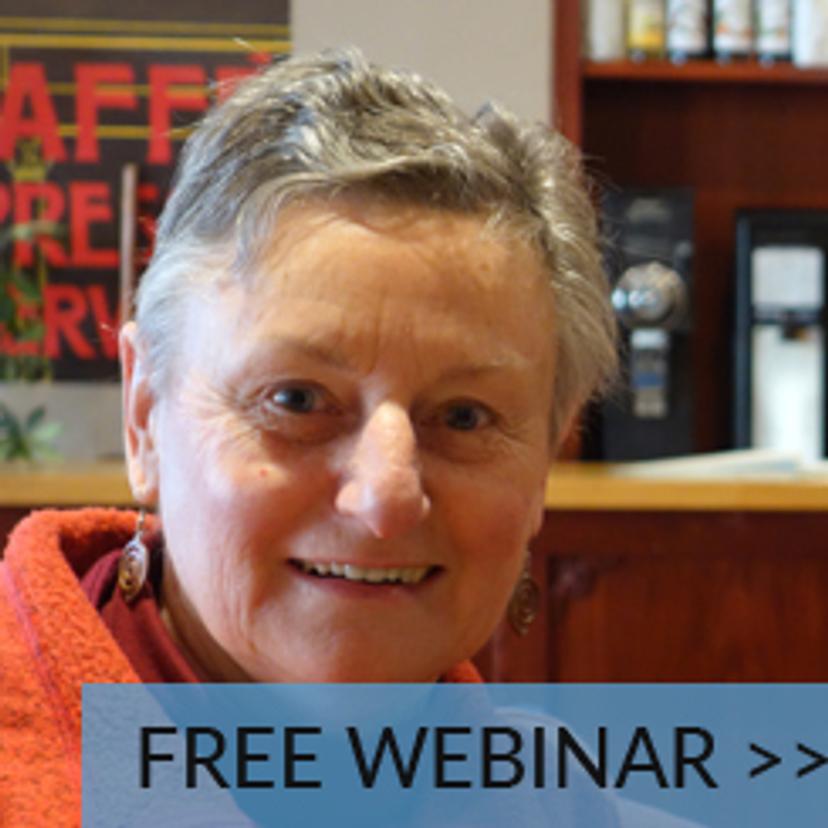
Q: How do you deal with the degeneracy of the NMR signals in the polypeptide backbone and side chains respectively?
JD: The primary problem in analyzing NMR spectra is precisely this problem, the degeneracy of the signals is what you must unravel in order to get the information out. This problem is exacerbated for disordered proteins because, unfortunately, what you see in the NMR spectrum is a very overlapped spectrum. Thus the first requirement to get over this is to have a high field magnet attached to a good spectrometer. That's what we have at Scripps and that's why we can look at these proteins. The second thing that you want to do is to use some isotope labeling; you can use specific labels or complete labeling. Another good thing is to perform is partial/full deuteration. This is a method which - because the methyl signals are very strong and well resolved - can be a very useful way of getting information on intrinsically disordered proteins and it can also be used for large proteins.
Q: By understanding the partly folded state of prion proteins, how did this assist in the treatment of prion induced diseases?
JD: That's a very good question. We did a lot of work on the prion protein, but the problem is that therapeutics are very difficult to design for these sorts of proteins. In fact, what happens in prion disease is that the folded domain of the protein partially unfolds, and amyloid is formed. It's very difficult to think of ways to stop that. At one point, we targeted it with copper ions in solution, but this is very difficult because you have to think of what you're going to introduce into a person when making a therapy for the disease. The main reason why we want to understand the folded and unfolded proteins business is that we may be able to design an overall therapeutic treatment, rather than trying to think about small molecules.
Q: Did you encounter exchange broadening among the fumbles in IDPs and, if you did, how do you deal with them?
JD: I talked about that to some extent, before. In fact, depending on the system, the exchange broadening can be quite horrible. One of our problems in one of the systems that we've been looking at recently is that the NMR structure was terrible. We tried very hard to overcome this by cutting the sequence up, using very high magnetic fields and so forth, but never really could get a very good handle on what was going on in the solution. Thus, the graduate student that was working on it said, "well, I'm going to see if I can crystallize this." Nobody in our lab had been able to crystallize anything but - lo and behold - she got crystals! We basically try to work with whatever we can get, to obtain the information. In this case, it happened to be a crystal structure because the NMR broadening was incredibly recalcitrant, and we couldn't get around it even with our best techniques.
Prof. Jane Dyson discusses her work with The Scientists' Channel>>
Q: As shown by your HSQC TAZ1 binding in selective and competitive in a complex cellular environment, do we expect a similar binding affinity?
Jane: Yes, I think we do. The cellular environment is very crowded, as you know. Basically, what we're looking at here is competition for the TAS1 domain or for the CBP. The CBP is a transcriptional coactivator that is basically the part of the mechanism of transcription that is limited in the cell. So, the ligands compete for it. In the case of TAS1, what happens is that the HIF-1-alpha has got to bind to the TAS1 very rapidly and very completely, in order to turn on the hypoxia genes quickly, before the cell dies of hypoxia. However, this is a very dangerous situation and so what happens is that cyto-2 comes along and takes it off in a very complete and rapid manner in order not to kill the cell with kindness by overdoing the hypoxia response.
Check out the dedicated NMR channel on The Scientists' Channel>>