AAV vectors in gene therapy clinical trials
Guest editorial article by Vikas Singh, PhD, and Thomas Beadnell, PhD, from Eurofins
6 Nov 2024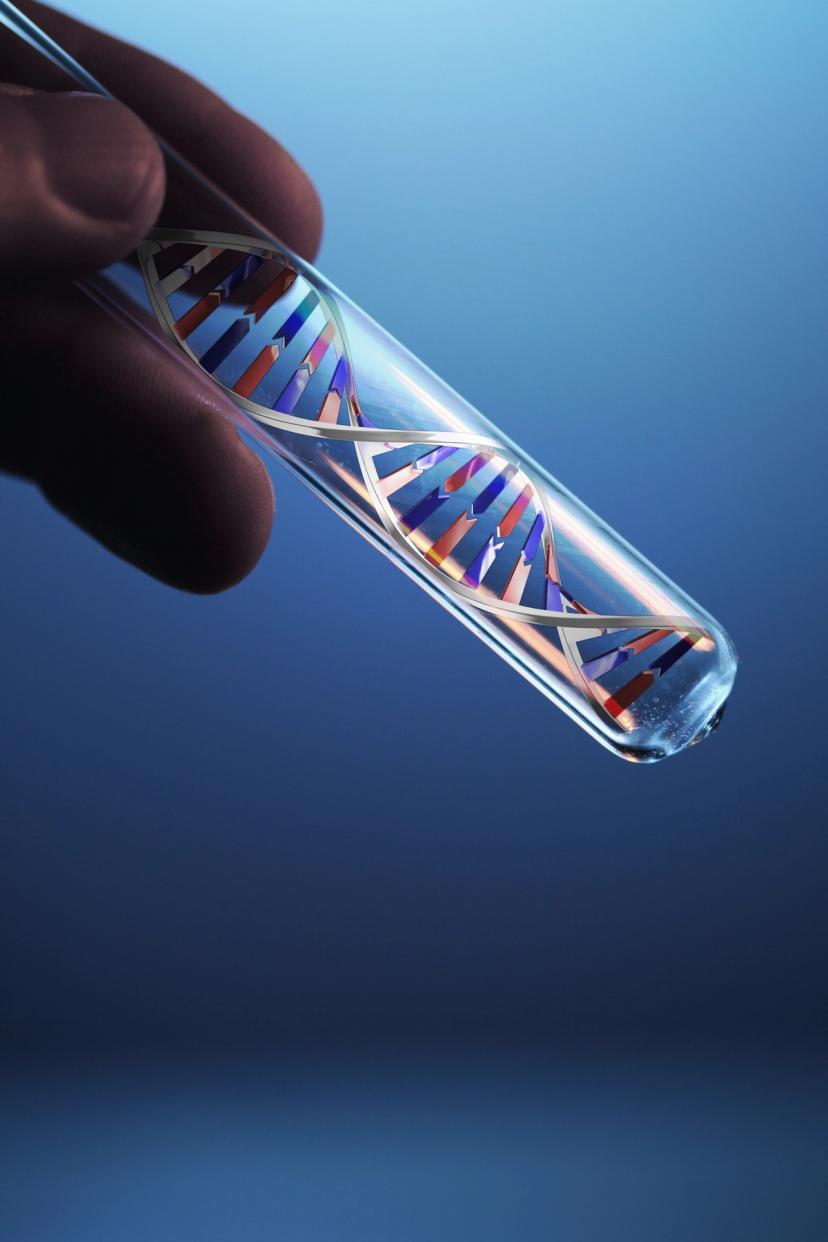
Novel gene therapy approaches provide successful treatment options to patients by delivering genetic material to replace, disrupt, or compensate flawed proteins. The primary mechanisms for delivering genetic material include viral delivery vectors (Lentivirus, Adenovirus, and Adeno-Associated Virus (AAV), with AAV vectors showing an increased utilization in gene replacement therapies 1.
In 2017, a major milestone was achieved with the first FDA approval of an AAV vector therapy (Luxturna) for gene replacement, which further highlighted AAV gene delivery as a promising therapeutic strategy, in part due to its improved safety profile over Lentiviral and Adenoviral vectors and tissue specificity. Lentiviral and Adenoviral based gene therapy vectors pose safety concerns associated with pathogenicity and vector integration. However, due to AAV’s reliance on helper viruses for replication, AAV provides a safer alternative, decreasing the likelihood of vector integration and pathogenicity 2.
Challenges of AAV therapy: Pre-existing immune responses
Despite the success of AAV-based gene therapy, a major challenge is immunity against the vector. Immune responses can be existing or induced and include neutralizing antibodies that prevent transgene integration, and/or recognition through major histocompatibility complex I (MHC I) or MHC II presentation that can signal leading to clearance of transduced cells by CD8+ T cells 3.
To ensure the success of gene therapy trials, it is crucial to navigate the challenges posed by both humoral and cellular immune responses. A growing body of research emphasizes the importance of understanding pre-existing humoral immunity in AAV-based clinical trials, as this knowledge can significantly impact gene expression outcomes 4,5. However, our understanding of cellular immunity in the context of AAV therapy remains more limited. Traditionally enzyme-linked immunosorbent assay (ELISA) has been the predominant readout for understanding patient pre-existing immunity for inclusion/exclusion criteria, however as our understanding of the importance of cellular immunity in gene therapy success evolves, the enzyme-linked immunosorbent assay (ELISpot) assay is increasingly important for the evaluation of pre-existing and induced T cell immunity.
ELISpot technology
The ELISpot assay is a powerful tool for detecting low frequency immune cells, focusing on antigen specific T cell or B cell responses. The ELISpot assay measures T cell activation through the incubation of peripheral blood mononuclear cells (PBMC) with peptides. The peptides are processed by antigen presenting cells and presented to T cells via MHC, enabling activation of memory and effector T cells. The assay utilizes plates coated with cytokine-specific antibodies that capture secreted cytokines at the sites of T cell activation, creating distinct spots corresponding to each activated T cell 6. In gene therapy trials, the peptides would be specific to the viral vector and/or transgene.
ELISpot assay validation
When used to support clinical trials ELISpot assays must be validated appropriately to the context of use. The validation parameters typically will include Precision, Sensitivity, Linearity, Specificity, Robustness, and Stability. The accuracy assessment would be more difficult to evaluate due to a lack of standard material 7.
A key requirement for completing an analytical validation is the availability of donor control samples. Generic control peptides can give insight into the overall health of the PBMC. Typically, the cells are activated through T cell receptor (TCR) crosslinking, to determine their activation potential. Common generic controls include phytohemagglutinin (PHA) and staphylococcal enterotoxin b (SEB) 8.
In order to confirm that an individual’s T cells are capable of responding to antigen presented by APC, peptide pools of common antigens are used as a control. For example, the CEF pool that includes peptides from CMV, EBV, and influenza. The CEFT pool adds tetanus peptides, while the CEFSX includes SARS-CoV-2 peptides 8. The CERI pool expands further to cover respiratory syncytial virus (RSV) and is designed to represent over 90% of distinct epitope combinations across five ethnic groups 9. A limitation of this approach is that the commercially available peptide pools are designed for presentation by HLA alleles prevalent in the Caucasian population and thus not relevant to other ethnic groups 9.
Both generic and TCR specific positive controls are crucial for ensuring the assay's reliability and accuracy, allowing for comparison with historical data to quickly identify deviations and troubleshoot throughout testing.
Negative controls are equally important, providing a baseline background signal to prevent the reporting of false positives. They often use media with the same peptide solvent concentration as the test wells. These controls might also include non-reactive peptides like human ß-actin or virus-based peptides for unexposed individuals (e.g., HIV peptides in HIV-negative people). However, virus-based peptides can cause issues if they react with previously encountered antigens, leading to higher-than-expected T-cell responses.
AAV specific controls and clinical validation
In addition to the analytical method validation described above, clinical validation is essential to interpret the utility of the ELISpot assay in monitoring patient responses during gene therapy clinical trials. This process assesses the validity of the cut-point, precision and determines the limits of the assay.
In many gene therapy studies, the absence of immunity to target antigens in the general population makes it difficult to identify donor controls with memory T cell responses to the vector or transgene. That said, with therapies using AAV vectors this is less of a problem given the prevalence of AAV infections.
The presence of AAV specific T cells in the general population highlights the importance of the ELISpot assay mediated identification of pre-existing immunity in patients, enhancing clinical trial screening. Contrary to the challenges associated with avoiding pre-existing immunity, the presence of healthy donors with AAV-specific T cell responses can help facilitate the development of a more specific and robust ELISpot assay, improving confidence in patient responses, refining the assay sensitivity, and providing a semi-quantitative readout.
The semi-quantitative AAV response data is crucial for understanding immune responses before and during trials, potentially leading to improved safety and efficacy data. However, as vector technologies evolve and AAV vectors are designed to mask similarities to wild type AAV and avoid immune recognition 2,10, it may become increasingly more challenging to identify positive responding donors to use in assay development/validation and as controls throughout the clinical trial. However there is a silver lining, as despite there being a lack of donor control material which poses a challenge to assay development, it does bring hope for more successful gene replacement therapies that are able to avoid AAV specific pre-existing immunity.
Conclusion
In summary, while AAV vectors represent a significant advancement in gene therapy, challenges related to immune responses must be carefully navigated to enhance therapeutic efficacy. Understanding both humoral and cellular immunity is crucial, and tools like the ELISpot assay provide valuable insights into T cell responses. The ongoing development and validation of these assays will play a pivotal role in the future success of AAV-based gene therapy, ultimately improving patient outcomes.
References
1. Arabi F, Mansouri V, Ahmadbeigi N. Gene therapy clinical trials, where do we go? An overview. Biomed Pharmacother. 2022 Sep;153:113324. Epub 2022 Jun 29. PMID: 35779421.
2. Wang JH, Gessler DJ, Zhan W, Gallagher TL, Gao G. Adeno-associated virus as a delivery vector for gene therapy of human diseases. Signal Transduct Target Ther. 2024 Apr 3;9(1):78. PMID: 38565561
3. Ronzitti G, Gross DA, Mingozzi F. Human Immune Responses to Adeno-Associated Virus (AAV) Vectors. Front Immunol. 2020 Apr 17;11:670. PMID: 32362898
4. Masat E, Pavani G, Mingozzi F. Humoral immunity to AAV vectors in gene therapy: challenges and potential solutions. Discov Med. 2013 Jun;15(85):379-89. PMID: 23819952.
5. Verdera H.C., Kuranda K., Mingozzi F. AAV Vector Immunogenicity in Humans: A Long Journey to Successful Gene Transfer. Mol. Ther. 2020; 28:723–746.
6. J., Kalyuzhny, A.E. (2018). Essential Controls for ELISpot Assay. In: Kalyuzhny, A. (eds) Handbook of ELISPOT. Methods in Molecular Biology, vol 1808. Humana Press, New York, NY. https://doi.org/10.1007/978-1-4939-8567-8_3
7. Handbook of ELISpot- 2024. An Overview of Peptides and Peptide Pools for Antigen-Specific Stimulation in T-Cell Assays Karsten Schnatbaum, Pavlo Holenya, Sebastian Pfeil, Michael Drosch, Maren Eckey, Ulf Reimer, Holger Wenschuh, and Florian Kern.
8. Francesca Dapporto, Domiziana De Tommaso, Camilla Marrocco, Pietro Piu, Claudia Semplici, Giulia Fantoni, Ilaria Ferrigno, Giulia Piccini, Martina Monti, Francesca Vanni, Ilaria Razzano, Ilaria Manini, Emanuele Montomoli, Alessandro Manenti. Validation of a double-color ELISpot assay of IFN-γ and IL-4 production in human peripheral blood mononuclear cells, Journal of Immunological Methods, Volume 524.
9. Lehmann AA, Reche PA, Zhang T, Suwansaard M, Lehmann PV. CERI, CEFX, and CPI: Largely Improved Positive Controls for Testing Antigen-Specific T Cell Function in PBMC Compared to CEF. Cells. 2021 Jan 27;10(2):248. doi: 10.3390/cells10020248. PMID: 33514016; PMCID: PMC7911306.
10. Naso MF, Tomkowicz B, Perry WL 3rd, Strohl WR. Adeno-Associated Virus (AAV) as a Vector for Gene Therapy. BioDrugs. 2017 Aug;31(4):317-334. PMID: 28669112