Frozen Assets – An Expert Guide to Biobanking
23 Dec 2014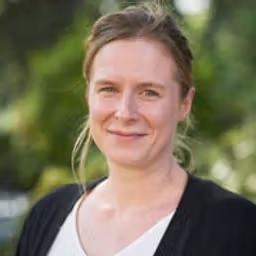
Biobanks may hold the key to quantum leaps in our ability to advance human health, but to unleash their full potential, sample collection, transport and handling procedures must be standardized.
By Eric J. Kunkel and Rolf O. Ehrhardt, BioCision LLC, San Rafael, CA
More than 60 years ago, scientists discovered that mammalian cells, with care and proper formulation, could be frozen, and subsequently revived with essentially no loss in function1. A new science was born – cryopreservation. Decades later, practically all industries that deal with cells or tissues make use of cryopreserved biological materials. The need for such materials has increased dramatically, in step with technological advances in areas such as assisted reproduction, genome sequencing, personalized medicine, stem cell research and transplantation science. But where do scientists get sufficient quantities of these materials? Enter the biobank.
What Exactly is a Biobank?
Biobanks are, by definition, repositories that store biological samples, usually at ultra-low temperatures. Some definitions restrict the term to refer to storage of samples of human origin, but others include animal, plant and single-cell organism specimens as well. Most importantly, the samples are annotated with relevant information about their origin, harvest procedure and storage conditions. Biobanks have become an important resource for scientists worldwide, and are now regarded as the foundation of personalized medicine2,3.
Types of Biobanks
Biobank classification is almost as diverse as the samples they store. Biobanks can be separated by owner (such as a government, research institute, or company), by location, or by the type of stored samples (such as tumor tissues, organs, bacterial cells, etc.). Based on the downstream use of the stored materials, biobanks of human samples can be broadly characterized as either disease-based or population-based. Disease-based repositories are often found at or near a hospital setting. The majority of biospecimens are from diseased individuals and may include blood components (plasma, white blood cells) and/or tumor cells and tissue biopsies. Population-based biobanks, on the other hand, generally exist in an independent setting and usually store samples from volunteers across large cross-sections of a general population4.
Processes that Affect Sample Quality
Sadly, not all material stored in biobanks is of comparable quality. Variations in sample processing and handling result in differences in subsequent cell and tissue viability and functionality, and also affect biomarker stability. Moreover, different sample types can be particularly sensitive to specific handling protocols, so it is imperative that scientists pay very close attention to the way the samples are treated. Several processes of particular importance to sample quality are discussed below.
Collection and Cryopreservation
Sample collection needs to be painstakingly controlled. During sample collection and preparation, the researcher should follow accurate guidelines and procedures from carefully drafted manuals, protocols and SOPs.
The overall goal of the subsequent freezing process is maintenance of biological fluid, cell, or tissue integrity. Several approaches exist for preserving sample integrity during freezing: (i) vitrification, i.e. very rapid freezing of biological materials, (ii) flash-freezing, and (iii) controlled-rate freezing. The choice of freezing procedure generally depends on the sample type to be frozen. Vitrification may better maintain functionality of whole organs and biological systems such as oocytes and embryos5,6 while flash-freezing is ideal for biological fluids (serum, urine). During preservation of cells or tissues, cryoprotectants (such as sugars, glycerol or dimethyl sulfoxide) are used to prevent intracellular ice crystal formation.
During slow-rate freezing, a temperature decrease of -1˚C / min optimally retains cellular integrity and function for most cell types7. A precisely controlled freezing rate can be obtained in high-performance programmable freezers, however, more cost-effective portable solutions are available that are as precise as these freezers. Such devices are now incorporated in clinical trials8 and standard protocols9. Freezing protocols are continuously optimized and tailored for the exact type of biospecimen to be stored (for example 10,11).
Transport and Storage
Virtually all cryopreserved biospecimens can be transferred into liquid nitrogen storage (approximately -150˚C to -196˚C), although biofluid samples are quite stable at -80˚C. At -150˚C or lower, metabolic activity of live biosamples ceases, providing stable long-term storage. However, transport of specimens from the harvest laboratory to storage and from storage to end-users is an area where temperature fluctuations can cause potential variability in sample quality. Thus, maintenance of ultra-low conditions at all stages during transport is required. Usually, this is obtained with high-quality packaging and dry ice or liquid nitrogen in quantities sufficient to last during unforeseen delivery delays.
Thawing
While freezing processes garner attention, few recommendations exist for the thawing of biospecimens. Improper thawing can affect sample viability just as much as imprecise freezing procedures, justifying additional care in this regard, particularly for live cells and tissues. Cell death, or apoptosis, that occurs after thawing is largely a delayed effect of mechanical and chemical stresses during both the freezing and thawing processes. Inhibitors of apoptosis added post-thaw can mitigate the effects of this stress, resulting in higher cell survival rates7, but these inhibitors can have unwanted effects on cell function. A thawing process that is removed from personal judgment and human variability is ideal, and recent efforts have been made in designing instruments that can help standardize the thawing process12.
The need for Standardization
Variability in biobanking processes seriously impacts the reproducibility and validity of scientific findings on cryopreserved material13, signaling an urgent need for extensive standardization of all processes involved in banking frozen biospecimens14. Authoritative guidelines such as those published by the International Society for Biological and Environmental Repositories (ISBER)15 which are developed and accepted by a panel of leading scientists, should be implemented to maximize the incredible potential that biobanks hold. Furthermore, emphasis on proper education of all personnel involved in collection, handling, and storage of samples is necessary. Finally, record keeping and annotation about the sample source, quality and processing need to be standardized in a way that maintains confidentiality and follows consent requirements, but provides the optimal amount of information for the research community.
References
1 Polge, C. et al. Revival of spermatozoa after vitrification and dehydration at low temperatures. Nature 164, 666, doi:http://www.ncbi.nlm.nih.gov/pubmed/18143360 (1949).
2 Hewitt, R. E. Biobanking: the foundation of personalized medicine. Current opinion in oncology 23, 112-119, doi:10.1097/CCO.0b013e32834161b8 (2011).
3 Olson, J. E. et al. Biobanks and personalized medicine. Clinical genetics 86, 50-55, doi:10.1111/cge.12370 (2014).
4 Ehrhardt, R. O. et al. Playing it cool. European Biopharmaceutical Review, 40-44 (2014).
5 Li, Y. et al. Comparison of three methods for cryopreservation of human embryonic stem cells. Fertility and sterility 93, 999-1005, doi:10.1016/j.fertnstert.2008.10.052 (2010).
6 Smith, G. D. et al. Prospective randomized comparison of human oocyte cryopreservation with slow-rate freezing or vitrification. Fertility and sterility 94, 2088-2095, doi:10.1016/j.fertnstert.2009.12.065 (2010).
7 Bissoyi, A. et al. Targeting cryopreservation-induced cell death: a review. Biopreservation and biobanking 12, 23-34, doi:10.1089/bio.2013.0032 (2014).
8 Scheppke, L. in Sampling Science Vol. 2014 (ed Thompson, M. L.) (2014).
9 Ramos, T. V. et al. Standardized cryopreservation of human primary cells. Current protocols in cell biology / editorial board, Juan S. Bonifacino ... [et al.] 64, A 3I 1-8, doi:10.1002/0471143030.cba03is64 (2014).
10 Katkov, II et al. DMSO-Free Programmed Cryopreservation of Fully Dissociated and Adherent Human Induced Pluripotent Stem Cells. Stem cells international 2011, 981606, doi:10.4061/2011/981606 (2011).
11 Miyazaki, T. et al. Cryopreservation of human pluripotent stem cells: a general protocol. Methods in molecular biology 1235, 97-104, doi:10.1007/978-1-4939-1785-3_9 (2015).
12 Standardized thawing using breakthrough solid-state technology. BioCision. ThawSTAR Automated Cell Thawing System
13 Barnes, R. O. et al. Influence of evolution in tumor biobanking on the interpretation of translational research. Cancer epidemiology, biomarkers & prevention : a publication of the American Association for Cancer Research, cosponsored by the American Society of Preventive Oncology 17, 3344-3350, doi:10.1158/1055-9965.EPI-08-0622 (2008).
14 Esmon, A. Standardization Will Enhance Sharing Between International Biobanks. Accelerating Science (2014).
15 Best practices for Repositories: Collection, Storage, Retrieval, and Distribution, of Biological Material for Research. ISBER 3rd Edition, 2012
Rolf Ehrhardt, MD, PhD - President and Chief Executive Officer
Dr. Rolf Ehrhardt co-founded BioCision in 2007, after more than 25 years of experience in leadership and innovation in medical research and product development. Prior to growing BioCision into the leading provider of temperature-sensitive sample and drug handling solutions, he was responsible for the early (phase 1a) clinical development of a novel HCV protease inhibitor at Intermune. Before that, Dr. Ehrhardt was the vice president of preclinical development at Corgentech. He was the founding president of BioSeek, where he raised close to $20 million in private financing. Prior to founding BioSeek, Dr. Ehrhardt led a scientific group focused on new target discovery and immuno-therapeutics development at Protein Design Labs (now PDL BioPharma Inc.). Dr. Ehrhardt was a Deutsche Forschungsgemeinschaft (DFG: German Research Foundation) and Fogarty Fellow at the National Institutes of Health, where his focus was on mucosal immunology and inflammatory bowel disease. He has authored more than 40 peer reviewed publications and holds multiple patents. Dr. Ehrhardt earned his M.D. and Ph.D. with distinction from the Technical University of Munich, Germany.
Eric J. Kunkel, PhD - Senior Vice President, Research and Development
Dr. Kunkel has over 12 years of experience in biotechnology including executive positions in R&D, entrepreneurship and start-ups, and consulting for technology and diagnostics companies. As an entrepreneur, he co-founded Ascellna Life Science Group, a business development and commercialization company for small companies with innovative technologies and Extend Biopharma, a start-up company developing a chemoenzymatic technology for creating next generation biologics. As VP, Assay Development and Screening at Catalyst Biosciences, Inc., Dr. Kunkel led the optimization of the company’s Alterase technology platform, which successfully generated multiple development candidates. Dr. Kunkel has published thirty-seven articles in peer-reviewed journals and has contributed to multiple patents and patent applications. He holds a B.Sc in Chemical Engineering from the University of Notre Dame, M.S. and Ph.D. degrees in Biomedical Engineering from the University of Virginia, and was a postdoctoral fellow at Stanford University.